Why are there so many living lights in the oceans?
The answer to this question lies in understanding the unique aspects of the marine visual environment, which shaped their evolution.
Water dramatically alters sunlight and creates gradients of light and color very different from what we are accustomed to on land. Immediately upon passing through the interface from air to water we are aware of a change in the color.
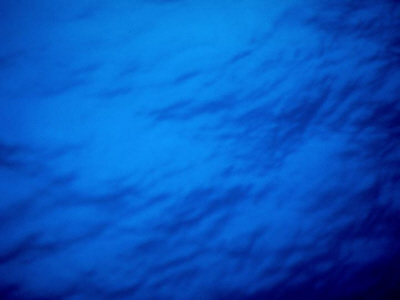
Figure 17 - Filtering effects of seawater. Photo credit: Tom Smoyer Harbor Branch Oceanographic Institution.
Everything looks blue underwater because water acts like a filter, attenuating certain colors more than others. The intensity of the light also changes dramatically. In clear ocean water light decreases 10 fold for every 75 m that you descend. This exponential attenuation of light with depth has a profound influence on where and how plants and animals live in the ocean.
Only in the thin surface layer, known as the epipelagic zone, is there enough light for photosynthesis. Below these sunlit surface waters is a twilight region known as the mesopelagic zone. In this zone, which extends from 200 m down to about 1000 m, there is enough available light for vision. However, throughout much of this depth range the light is too dim for animals to see much more than faintly resolved shapes and shadows. Imagine trying to find someone you know in a very dark theater. A flashlight would help. The same is true in the mesopelagic zone where animals depend on bioluminescence as their primary means of communication. Living lights dominate this twilight realm. In fact, if you dragged a net through the mesopelagic zone you would find that approximately 80-90% of all the fish, shrimp and squid that you caught would be bioluminescent.
Sunlight doesn’t penetrate below 1000 m but there are still animals with eyes – eyes for seeing bioluminescence. In the bathypelagic zone, which extends from the bottom of the mesopelagic zone (1000 m) down to the average bottom depth of the ocean (4000 m), approximately 25% of the animals caught in nets are luminescent.
Below these depths are the deep valleys, which cover the depth range between 4000 and 6000 m and are known as the abyssopelagic zone and the deepest canyons and trenches, which extend down as far as 10,915 m and are known as the hadal zone. Bioluminescence occurs even at these depths, but with increasing depth, there are fewer and fewer organisms, both bioluminescent and non-bioluminescent.
In the upper regions of the oceans, where sunlight penetrates one of the driving forces in the evolution of animals in these waters has been their need to hide from each other. During the course of evolution as the ocean filled up with ever swifter and nastier predators animals had to be able to outswim predators or evolve some way to hide. In a world without hiding places, this presents a difficult challenge. Some animals have met this challenge by becoming invisible (Figure 18).
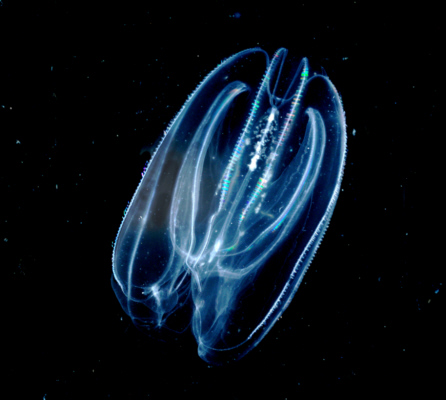
Figure 18 The comb jelly (ctenophore) Bolinopsis infundibulum is so transparent you can see what it had for dinner. Edith A. Widder Harbor Branch Oceanographic Institution.
Many others have met it by hiding in the dark depths during the day and only coming up to feed in food-rich surface waters under cover of darkness. This results in the largest animal migration pattern on the planet, known as vertical migration. Most vertical migrators are bioluminescent because being able to produce light has proven to be a powerful evolutionary benefit to visual animals living in perpetual dim twilight — either very dim filtered sunlight at depth during the day or dim moonlight and starlight near the surface at night. This may help account for why bioluminescence has appeared independently so many times (currently estimated at about 30 different times) during evolutionary history. When a number of organisms arrive at the same solution to a problem (like how to survive in the dark) this is known as convergent evolution, and is evidence for the tremendous survival advantage of the trait.
Why do animals make light?
The reason that there are so many living lights in the ocean is that their lights can serve as important aids to survival. To survive an animal must 1) eat 2) not be eaten and 3) reproduce. In dim light environments bioluminescence can help with all these vital functions.
If you are looking for your dinner in the dark a flashlight can be a very handy tool. It’s not just flashlight fish that use built-in flashlights. This loosejaw fish (Figure 19), for example has a built in flashlight. In this case there is no symbiotic relationship with bacteria, because the fish manufactures its own light-producing chemicals.
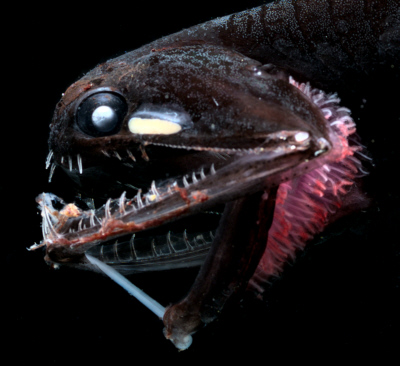
Figure 19 The loosejaw fish, Photostomias guernei, has a light organ under each eye that it can use for seeing in the dark. These light organs are non-bacterial and are under neural control. Edith A. Widder Harbor Branch Oceanographic Institution.
Anglerfish are not the only ones to use lures. Viperfish also have a bioluminescent lure on the end of a long fishing pole (Figure 20). As with the anglerfish, the fishing pole is a modified dorsal fin ray, but unlike the anglerfish the viperfish synthesizes its own bioluminescent chemicals.
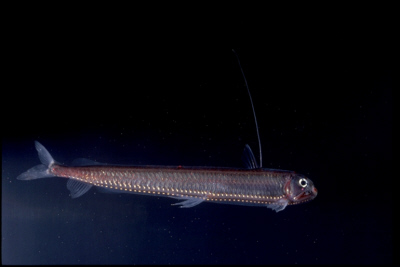
Figure 20 - The viperfish Chauliodus sloani, has a non-bacterial light organ at the tip of its modified dorsal fin ray, that it can use as a lure. Photo credit: Edith A. Widder Harbor Branch Oceanographic Institution.
There are also fish that use luminescent lures on the end of a chin barbel. These come in a whole range of sizes from very long (Figure 21), to very short (Figure 22).
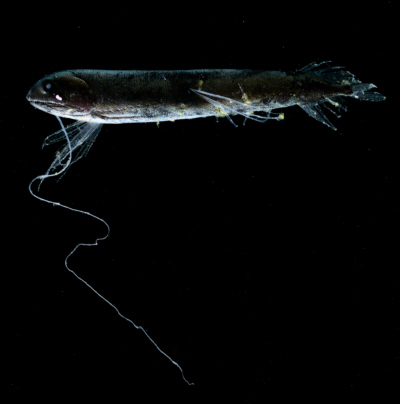
Figure 21 - The highfin dragonfish, Bathophilus nigerrimus, has one of the longest luminescent chin barbels known. Photo credit: Edith A. Widder Harbor Branch Oceanographic Institution.
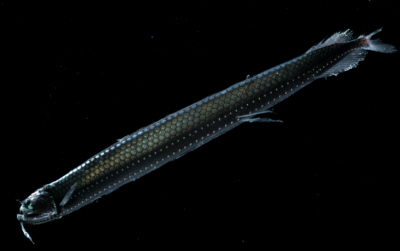
Figure 22 The blackbelly dragonfish, Stomias brevibarbatus, has one of the shortest chin barbels. Photo credit: Edith A. Widder Harbor Branch Oceanographic Institution.
Bioluminescence can also come in pretty handy if you are trying to locate a mate in the dark. The reason for the very elaborate shapes of the luminescent lures of anglerfish was once thought to be so they would attract different kinds of prey, but when it was discovered that all these fish seemed to subsist on pretty much the same diet, that theory needed to be revised. Now it is believed that males use the shape of the female’s lure to identify a female of his species. Anglerfish males are dwarf males, much smaller than the females and lacking visible means of self-support, like a luminescent lure or teeth. To survive he must locate an appropriate female and latch on for life. He then becomes a permanent source of sperm for the female (Figure 23). This is an extreme example of sexual dimorphism. Other examples include fish where males and females have different light organs or different sized light organs.
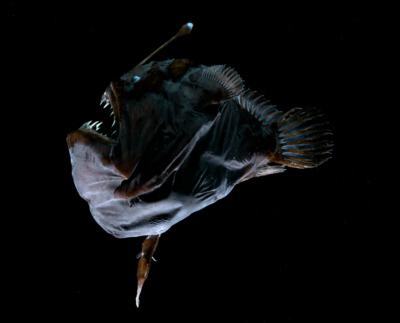
Figure 23 A female anglerfish with attached, parasitic, male. Photo credit: Edith A. Widder Harbor Branch Oceanographic Institution.
Bioluminescence may also function to deter predators. Just as a squid or octopus can release an ink cloud to distract a predator, many bioluminescent animals can release their luciferin and luciferase into the water to distract or temporarily blind a predator. There are shrimp that can do this (Figure 24), as well as squid, fish and jellyfish. The eyes of many mesopelagic predators are large and optimized for seeing the faintest hint of light and they don’t have eyelids or stop-down irises, so a face full of blinding light is a pretty effective form of distraction.
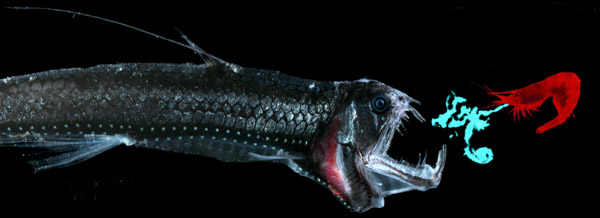
Figure 24 - The deep-sea shrimp Acanthephyra purpurea, vomits light into the face of a predator and then backflips away into the darkness. Photo credit: Edith A. Widder Harbor Branch Oceanographic Institution.
With so many eyes in the depths tuned to detect a flash of light that might reveal potential prey, there is another very effective defense known as a burglar alarm, which many animals employ. Once an animal is caught in the clutches of a predator, very often its only hope for escape is to attract the attention of a larger predator that may attack their attacker. This is the purpose of fear screams that some animals produce when caught by a predator and it is also the purpose of the flashing lights and blaring alarms that humans use on their homes and cars. (In the case of humans, the larger predators that these alarms are meant to attract are the police.) Because their purpose is to attract attention burglar alarms are often spectacular
See Video clip #2 Deep-sea jellyfish.
Video clip #2 The deep-sea scyphozoan jellyfish, Atolla wyvillei, seen first in white light, from the submersible and then with the lights out, revealing its remarkable burglar alarm display. Photo credit: Edith A. Widder Harbor Branch Oceanographic Institution
Burglar alarms are also the reason for the bioluminescent flashing of dinoflagellates. It has been demonstrated in laboratory experiments that copepods, the primary grazers on bioluminescent dinoflagellates, reduce their grazing on bioluminescent as compared to non-bioluminescent dinoflagellates. In order to address the possibility that bioluminescent dinoflagellates differ from non-bioluminescent dinoflagellates in some fundamental way that could be the real cause of this reduction, these experiments took advantage of the fact that many dinoflagellates exhibit a circadian rhythm, and are only luminescent in their night phase. It is believed that copepods reduce grazing on bioluminescent dinoflagellates in order to avoid drawing the attention of visual predators.
Another very important use of bioluminescence in the mesopelagic zone is as camouflage. Animals swimming in these twilight depths are easy targets for upward looking predators that search for telltale silhouettes against dim downwelling sunlight. In order to camouflage themselves some animals, such as sharks, are countershaded. They have a dark back, so if you look at them from above, they blend into the dark depths. And they have a white belly, so if you look at them from below, they blend in with the bright overhead light (Figure 25).
But at deeper depths there is less light to reflect off bright underbellies, so many animals have evolved a camouflage strategy known as counterillumination. Essentially this involves replacing the sunlight absorbed by their tissues with bioluminescence. It is a remarkably effective camouflage trick. Although, the individual photophores, or light organs arrayed across bellies of most counterilluminators may seem like a less than ideal camouflage (Figure 26), in fact, these work very well because of how rapidly light is scattered underwater. These spots blur and blend perfectly into the background of the downwelling light field. They not only match the color of filtered downwelling sunlight they also match the intensity. If a cloud passes over the sun dimming the background light the fish detects the change and adjusts its bioluminescence accordingly to maintain its camouflage.
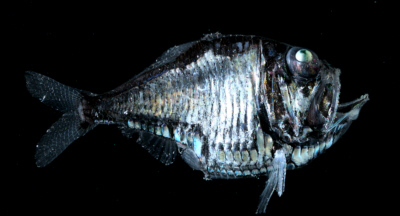
Figure 26(A) The hatchetfish, Argyropelecus affinis, uses bioluminescence as a cloaking device to mask its silhouette. Photo credit: Edith A. Widder Harbor Branch Oceanographic Institution.
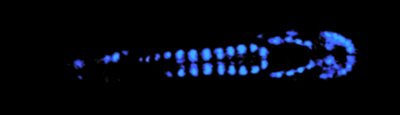
Figure 26(B) The hatchetfish, Argyropelecus affinis, uses bioluminescence as a cloaking device to mask its silhouette. Photo credit: Edith A. Widder Harbor Branch Oceanographic Institution.
Activity #4 In order to be able to see in dim light environments many mesopelagic organisms have eyes that are optimized for seeing in the dark. Human eyes can attain a rather remarkable degree of sensitivity if given sufficient time to dark-adapt (about 20 mins for full dark-adaptation). It is believed that there are deep dwelling fish that have as much as 10 times this sensitivity.This activity demonstrates how important low light sensitivity is for locating prey in the mesopelagic or twilight zone and how effective bright bioluminescence can be at temporarily blinding a predator.
The goal is to have students dark-adapt one eye and light-adapt the other and then directly compare their resolving power under dim light. The simplest way to accomplish this is to have students cover one eye with a black patch and then go about normal activities for about 20 mins in the light. At the end of this dark adaptation period, darken the room and have students try to read a very dimly illuminated reading chart or message on the wall with first their light-adapted eye and then their dark-adapted eye. For the dim illumination you can use a small flashlight with a blue filter or many layers of blue tissue paper over it, but try it yourself first, to make sure you have the light levels just right. The effect should be dramatic, with the chart being readable to the dark-adapted eye and essentially invisible to the light-adapted eye. You can also post some phosphorescent stars or other glow-in-the-dark decorations around the room, which will add to the effect.
What are some applications of living lights?
Bioluminescent chemicals extracted from living lights have proven to be very valuable tools for science. For example, photoproteins, which are complexes of luciferin and luciferase, have been extracted from jellyfish and their light production has been used as a very sensitive test for the presence of calcium (http://www.wsg.washington.edu/story/storyarchives/blinks.html).
One such photoprotein is aequorin, which was extracted from the jellyfish Aequorea victoria (Fig 27) (http://faculty.washington.edu/cemills/Aequorea.html).
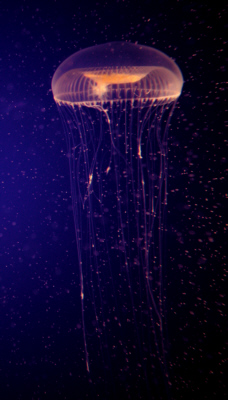
Figure 27 - The jellyfish Aequorea. Photo credit: Edith A. Widder Harbor Branch Oceanographic Institution.
Also extracted from this same jellyfish is the now more famous Green Fluorescent Protein or GFP. GFP is an accessory protein that accepts energy from a bioluminescent reaction and re-emits it as light of a longer wavelength (http://bmbiris.bmb.uga.edu/wampler/biolum/gfp/sld019.html). This protein has proven very valuable to genetic engineers who use it as an assay for protein expression. By attaching the gene for GFP to other genes, they can tell when and where those genes are expressed by tracking when and where green fluorescence appears in the cell.
Bioluminescent bacteria are used in bioassays to test for pollutants and toxins. In a healthy environment luminescent bacteria glow brightly but in the presence of unhealthy chemicals, their light goes out. The sensitivity of these assays is so high that these glowing bacteria can function like the canaries that miners used to use to detect carbon monoxide, before it reached lethal concentrations. The bacteria can reveal trace amounts of toxins before they reach lethal concentrations.
Because bioluminescence is so common in the oceans it is a useful tool for mapping organism distribution patterns. One of the toughest problems that marine biologists face is mapping the abundance and distribution of animals in this vast fluid environment. Traditionally, this has been accomplished by dragging nets behind ships. The problem with net sampling is that it provides only an integrated view of the volume sampled with no indication of how animals distribute themselves in space relative to each other. It is also very labor intensive and time consuming to analyze these samples, so much so that reports are often not published until years after the samples are collected. And finally, nets destroy the fragile gelatinous zooplankton, which recent studies with SCUBA, submersibles and remote operated vehicles (ROVs) have shown to be major players in the marine environment. Many animals can be identified by the spatial and temporal properties of their bioluminescent displays and this capability has been used to develop 3D maps of plankton distribution patterns.
From : Classroom Exploration of the Ocean, College of Exploration, Virtual Teacher’s workshop May 04, 2003
Leave a Reply